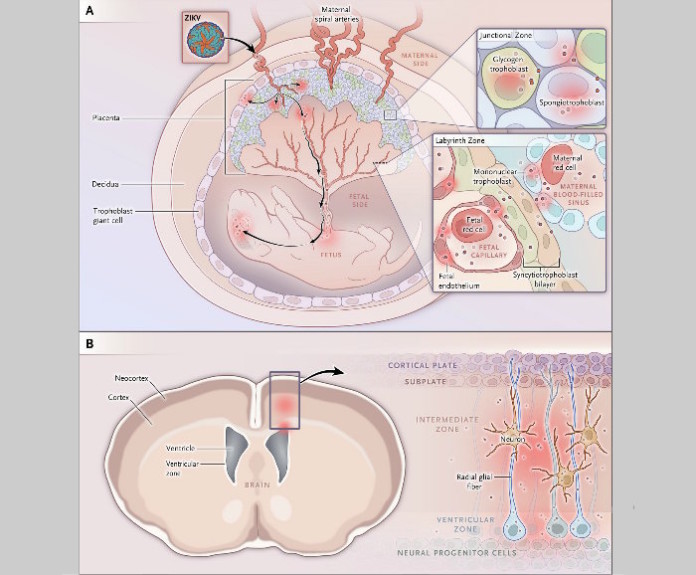
There were few studies of Zika virus (ZIKV), a flavivirus, until this past year, when large epidemics in the Americas were accompanied by unexpectedly severe clinical manifestations. Infection in pregnant women has emerged as a major global concern because of its linkage to congenital abnormalities including microcephaly, spontaneous abortion, and intrauterine growth restriction. In addition, ZIKV infection in other age groups has been associated with severe neurologic disease and the Guillain–Barré syndrome.
Transmission cycles between humans and Aedes aegypti mosquitoes in urban settings can cause large-scale epidemics of ZIKV infection. Although mosquitoes clearly are the primary cause of ZIKV outbreaks, other modes, such as sexual transmission,3 have been reported. So far, all cases of sexual transmission of ZIKV have been from infected men to their partners. Viral persistence in the testes and semen has been described, and the window of sexual transmission remains uncertain, which has increased the apprehension about ZIKV infection during pregnancy.
The question of in utero transmission attained prominence as the emergence of ZIKV in Brazil and other parts of Latin America became associated with an increase in the number of cases of microcephaly. Data that support vertical infection and a causal role for ZIKV in the development of congenital malformations include the detection of ZIKV RNA or antigen in the amniotic fluid, the placenta, or the brain tissues of fetuses or infants in whom microcephaly is diagnosed after death in utero or soon after birth. Moreover, a prospective study involving ZIKV-infected pregnant women in Brazil showed that 29% of fetuses had gestational abnormalities including microcephaly and intrauterine growth restriction, which in a subgroup of cases resulted in fetal death. Apart from this evidence, the development of an in utero animal model of ZIKV transmission and infection was considered to be important in order to establish causality, satisfy the criteria for proof of teratogenicity, and provide a means for screening candidate vaccines and therapeutic agents.
Four recently published mouse-model studies have addressed the causal relationship between ZIKV infection in pregnancy and pathologic changes in fetuses. Three of the studies introduced different ZIKV strains into pregnant mice through peripheral inoculation routes (intravenous, intraperitoneal, or subcutaneous) and two studies inoculated ZIKV directly into the fetal brain. All the studies established ZIKV infection in fetuses with resultant injury to cells in the central nervous system; one study showed the cellular basis of transmission of ZIKV from maternal blood to the fetus.
Flaviviruses must overcome type I interferon–receptor signaling in order to replicate in cells; in part, ZIKV achieves this by inducing the degradation of the STAT2 signaling molecule (a messenger protein that is downstream of the interferon receptor) in human cells. Because ZIKV inefficiently promotes the degradation of mouse Stat2, Miner et al. used mice that were deficient in type I interferon–receptor signaling so that a sufficient level of viremia could be achieved to seed the placenta. This group inoculated a contemporary Asian ZIKV strain into the skin of pregnant mice early in gestation (at E6,5 equivalent to the first trimester of pregnancy in humans) and observed placental infection, injury, and insufficiency, a high rate of fetal resorption, and fetal brain injury and neuronal-cell death. These observations recapitulate findings in humans that occur in some ZIKV-infected women during the first and second trimesters of pregnancy. Moreover, this group identified ZIKV RNA and virus within trophoblasts and fetal endothelial cells in the placenta, findings that are consistent with a tropism for cells lining the maternal–fetal interface and a transplacental route of infection.
Cugola et al. inoculated pregnant immunocompetent SJL mice through an intravenous route with an extraordinarily high dose (1010 to 1012 plaque-forming units) of a Brazilian strain of ZIKV. Possibly, this dose allowed some of the virus inoculum to seed the placenta directly without requiring much replication in peripheral organs, where it would be expected to be inhibited by type I interferon. As in the findings of Miner et al., they observed intrauterine growth restriction that resulted in smaller pups at birth. Examination of the fetal and neonatal brains revealed a reduced number of cortical neurons and reduced cortical-layer thickness. Cellular analysis showed that neurons in the cerebral cortex, thalamus, and hypothalamus had evidence of deterioration. In addition, this group noted ocular abnormalities that were similar to those that have been described in humans.
Li et al. and Wu et al. injected contemporary Asian ZIKV strains directly into the lateral left ventricle of fetal brains at E13.5 (equivalent to the late second trimester of pregnancy in humans) and analyzed tissues before or after birth. Both groups observed a reduction in the number of cortical neural progenitors in the dorsal ventricular and subventricular zones, and this loss was associated with cell death. Wu et al. also injected ZIKV through an intraperitoneal route into pregnant immunocompetent mice at E13.5. They found that ZIKV could cause a transient viremia with seeding of the placenta in a subgroup of pregnant mice. Somewhat unexpectedly, they found ZIKV RNA in the dorsal ventricular zone of the fetal brain but in no other brain regions. They concluded that ZIKV can cross the fetal–placental barrier and specifically target the cortical neural progenitors of fetal mice.
Three of the four studies suggest that ZIKV gains access to the fetus during pregnancy after crossing the placental barrier, which is composed of different types of trophoblasts and ancillary cells. On the basis of the mouse models as well as recent studies of infection in primary human cells and tissue samples, investigators have found that ZIKV probably replicates in subgroups of trophoblasts, fetal endothelial cells, and Hofbauer placental macrophages. Access to these cell types may be enhanced by the binding of ZIKV to a cell-surface tyrosine kinase receptor called AXL. Early in pregnancy, ZIKV infection may lead to severe placental vascular damage and a reduction in fetal blood vessels and blood flow. Alternatively, ZIKV could cross the placental barrier without excessive damage and spread to the fetal brain, where it preferentially infects and injures neuronal progenitor cells. This outcome may be more typical of infection later in pregnancy because of enhanced interferon-λ–induced innate immunity in trophoblasts. Infection and death of neuroprogenitor cells could inhibit neuronal-cell differentiation, which would explain the cortical thinning, malformation of brain structures, and microcephaly that are observed during pregnancy in humans.
Investigators must consider the differences in morphologic, spatial, and temporal placentation and in utero brain development in rodents and humans. However, many elements of placental development and trophoblast function are similar in the two species. Although the time scale differs substantially, neuronal developmental processes in rodents and humans are remarkably parallel. Collectively, the findings from the studies in mice support the hypothesis that infection during pregnancy with contemporary ZIKV strains causes placental infection and injury and that ZIKV spreads to the fetal brain and kills neuronal progenitor cells, which probably contributes to the microcephaly and other congenital malformations that have been observed in human neonates. The new mouse models provide a foundation for defining mechanisms of pathogenesis and represent a resource for determining the effects of ZIKV strain variation on clinical manifestations and for testing candidate therapeutic agents and vaccines.
Authors: Indira U. Mysorekar, Ph.D., and Michael S. Diamond, M.D., Ph.D. DOI: 10.1056/NEJMcibr1605445
Source: NEJM